DNA Replication Mechanics: Why the Lagging Strand Synthesis is Discontinuous
Understand DNA replication: the basics
DNA replication is a fundamental biological process that ensure genetic information is accurately copied before cell division. During this intricate molecular dance, both strands of the parental DNA double helix must be duplicate. Nonetheless, the two result daughter strands are synthesized through unmistakably different mechanisms. While the lead strand is produce endlessly, the lagging strand is synthesized in a discontinuous fashion, create short DNA fragments that are afterward joined unitedly.
This difference in synthesis methods isn’t arbitrary but stem from the inherent constraints of DNA structure and the enzymes involve in replication. Understand why discontinuous synthesis occur on the lagging strand provide critical insights into the elegance of cellular machinery.
The directional constraint of DNA polymerase
The primary reason for discontinuous synthesis on the lagging strand is the directional limitation of DNA polymerase, the enzyme responsible for add nucleotides to create the new DNA strand. DNA polymerase can merely add nucleotides in the 5′ to 3′ direction. This directional constraint is a biochemical property of the enzyme that can not be overcome.
DNA’s double helix consist of two antiparallel strands, mean they run in opposite directions. One strand run in the 5′ to 3′ direction, while the complementary strand run 3′ to 5′. When these strands separate during replication, they create a replication fork that move along the DNA molecule.
At this replication fork, both strands must be replicate simultaneously. Since DNA polymerase can sole synthesize in the 5′ to 3′ direction, but the template strands run in opposite directions, a problem arises: one strand canbe synthesizede endlessly in the same direction as fork movement, while the other musbe synthesizedze against the direction of fork movement.
The leading strand: continuous synthesis
On the lead strand, DNA synthesis proceed swimmingly and endlessly. This is because the template strand run in the 3′ to 5′ direction relative to the movement of the replication fork. DNA polymerase can add nucleotides in its required 5′ to 3′ direction while the replication fork move forward moving, allow for uninterrupted synthesis.
The process begin with a short RNA primer synthesize by primate. DNA polymerase so extend this primer, endlessly add nucleotides to create the new lead strand. This synthesis occur in the same direction as the replication fork movement, allow for efficient and uninterrupted DNA production.
The lagging strand: discontinuous synthesis necessity
On the lagging strand, the situation is essentially different. The template strand run in the 5′ to 3′ direction relative to the replication fork movement. Since DNA polymerase can exclusively synthesize in the 5′ to 3′ direction, it must work against the direction of fork movement.
If DNA polymerase were to attempt continuous synthesis on this strand, it’d need to move backwards against the replication fork, which is physically impossible as the replication machinery move forward moving, endlessly expose new template DNA.
The solution to this directional dilemma is discontinuous synthesis. Quite than attempt to synthesize endlessly against the direction of fork movement, the replication machinery produces short segments ofDNAa callOkazakii fragments, name after their discoverer,Rajii Okazaki.
Okazaki fragments: the building blocks of the lagging strand
Okazaki fragments are short segments of DNA, typically between 1,000 to 2,000 nucleotides proficient in bacteria and 100 to 200 nucleotides in eukaryotes. Each fragment begin with aarnRNArimer synthesize by prprimatewhich provide the essential 3′ hydroxyl group that dnDNAolymerase require tostartingdd nucleotides.
As the replication fork move ahead, expose new template DNA on the lagging strand, primate sporadically synthesize new RNA primers. DNA polymerase so extend these primers in the 5′ to 3′ direction, create discrete Okazaki fragments. This process repeat multiple times as the replication fork progress, result in numerous short DNA segments along the lagging strand.
The discontinuous nature of lag strand synthesis allow DNA polymerase to maintain its 5′ to 3′ directionality while motionless replicate the entire lagging strand template. Each Okazaki fragment is synthesized in the 5′ to 3′ direction, but jointly they progress in the opposite direction of the replication fork movement.
Processing Okazaki fragments: create a continuous strand
Once Okazaki fragments are synthesized, they must be process to create a continuousDNAa strand. This processing involve several steps:
-
RNA primer removal:
The RNA primers that initiate each Okazaki fragment must be removed. This task isperformedm DNAdna polymerase i in prokaryotes or RNASase h and fen(( flap endonuclease )) in eukaryotes. -
Gap filling:
After primer removal, gaps remain in the DNA strand. DNA polymerase fill these gaps by extend the 3′ end of the adjacent Okazaki fragment. -
Fragment joining:
Eventually, the adjacent Okazaki fragments must be joined unitedly to create a continuouDNAna strand. This crucial step iperformedrmDNA dna ligase, which catalyze the formation of a phosphodiester bond between the 3′ hydroxyl group of one fragment and the 5′ phosphate group of the adjacent fragment.
Through this processing, the initially discontinuous lagging strand is convert into a continuous DNA molecule that’s identical to the lead strand in terms of integrity and information content.
The evolutionary perspective: why not evolve bidirectional DNA polymerase?
An interesting question arise: why hasn’t evolution produce a DNA polymerase that can synthesize DNA in both the 5′ to 3′ and 3′ to 5′ directions? Such an enzyme would eliminate the need for discontinuous synthesis on the lagging strand.
The answer lie in the chemistry of nucleotide addition. The reaction involve nucleophilic attack by the 3′ hydroxyl group of the growtDNAna strand on the phosphate of the incoming nucleotide. This chemistry powerfully favor synthesis in the 5′ to 3′ direction. Create an enzyme that could expeditiously catalyze synthesis in the opposite direction would require an essentially different reaction mechanism.

Source: tffn.net
Additionally, the discontinuous synthesis mechanism provide certain advantages. It allows for the removal ofRNAa primers and correction of any errors that might haveoccurredr during initial synthesis. Tcontributesbute to the remarkable accuracDNAf dna replication, with error rates adenine low as one mistake per billion nucleotides in some organisms.
The role of discontinuous synthesis in DNA repair
The mechanisms develop for process Okazaki fragment during lag strand synthesis have been repurposed by cells forDNAa repair. ManyDNAa repair pathways involve the removal of damaged nucleotides, creation of gaps, gap filling byDNAa polymerase, and seal byDNAa ligase — steps that mirror the processing ofOkazakii fragments.
This repurposing of enzymatic machinery for multiple cellular processes is a common theme in evolution, where exist mechanisms are adapted for new functions preferably than develop totally new systems.
Variations in different organisms
While the basic mechanism of discontinuous synthesis on the lagging strand is conserved across all domains of life, there be notable variations between prokaryotes and eukaryotes:

Source: technewshunt.com
-
Okazaki fragment size:
In bacteria, Okazaki fragments are typically 1,000 2,000 nucleotides proficient, while in eukaryotes, they’re lots shorter, ordinarily 100 200 nucleotides. This difference reflect the more complex chromatin structure in eukaryotes and different processing enzymes. -
Processing enzymes:
In prokaryotes, DNA polymerase I remove RNA primers and fill gaps, while in eukaryotes, this task is performed by a combination oRNASse h, fen1, andDNAa polymerase δ. -
Replication speed:
Bacterial replication proceed practically fasting than eukaryotic replication, with fork movement rates of about 1,000 nucleotides per second in bacteria compare to roughly 50 nucleotides per second in human cells.
These variations highlight how the fundamental process of discontinuous synthesis has been adapted to the specific needs and constraints of different organisms.
Implications for genetic disorders and cancer
Defects in the enzymes involve in lag strand synthesis and Okazaki fragment processing can lead to genetic instability, a hallmark of cancer and various genetic disorders. For example:
- Mutations in DNA ligase I can cause growth retardation, immunodeficiency, and increase sensitivity to DNA damage agents.
- Defects in fen1 have been associate with increase cancer susceptibility due to its role in maintain genome stability.
- Problems with DNA polymerase δ can lead to increase mutation rates and potentially contribute to cancer development.
Understand the molecular details of lag strand synthesis provide insights into these disorders and may suggest therapeutic approaches target specific steps in the replication process.
Conclusion: the elegant solution of discontinuous synthesis
The discontinuous synthesis of the lagging strand represent an elegant solution to the directional constraints impose by DNA structure and DNA polymerase biochemistry. Instead, than developan completely new enzyme capable of synthesize DNA in the opposite direction, cells have evolved a comple, butt efficient system of discontinuous synthesis follow by process to create a continuouDNAna strand.
This mechanism ensure accurate DNA replication while work within the constraints of molecular biology. The lagging strand synthesis process illustrates a fundamental principle in biology: natural systems oftentimes develop sophisticated workarounds for biochemical constraints sooner than basically alter the underlie chemistry.
The study of discontinuous DNA synthesis continue to provide insights into fundamental cellular processes and have implications for understanding genetic disorders, cancer biology, and potential therapeutic approaches targDNAdna replication. standsand as a testament to the remarkable complexity and efficiency of cellular machinery that evolvedolve over billions of years.
This text was generated using a large language model, and select text has been reviewed and moderated for purposes such as readability.
MORE FROM couponito.com
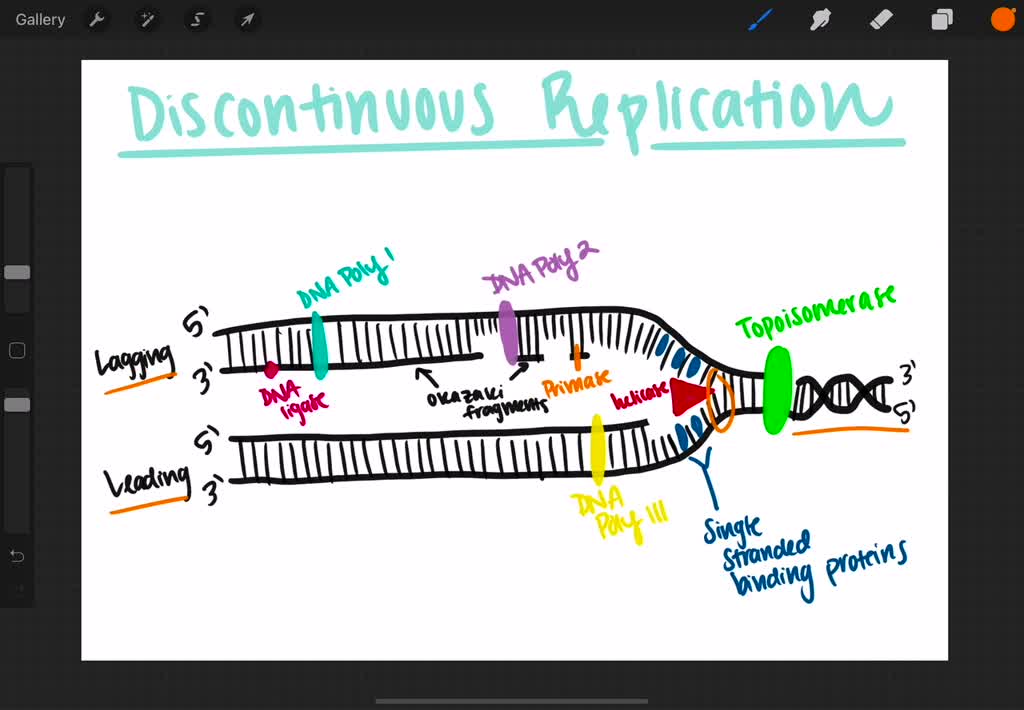
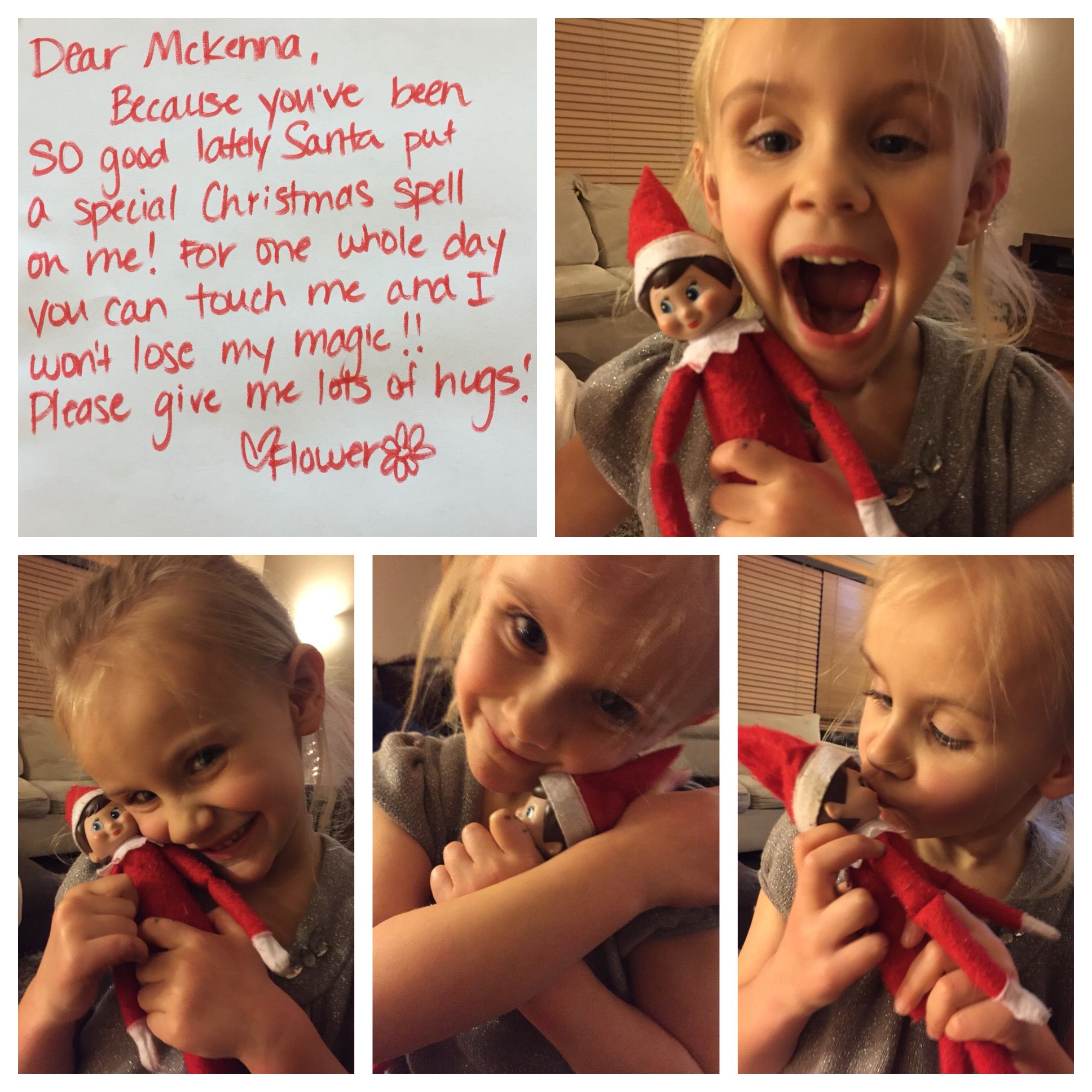
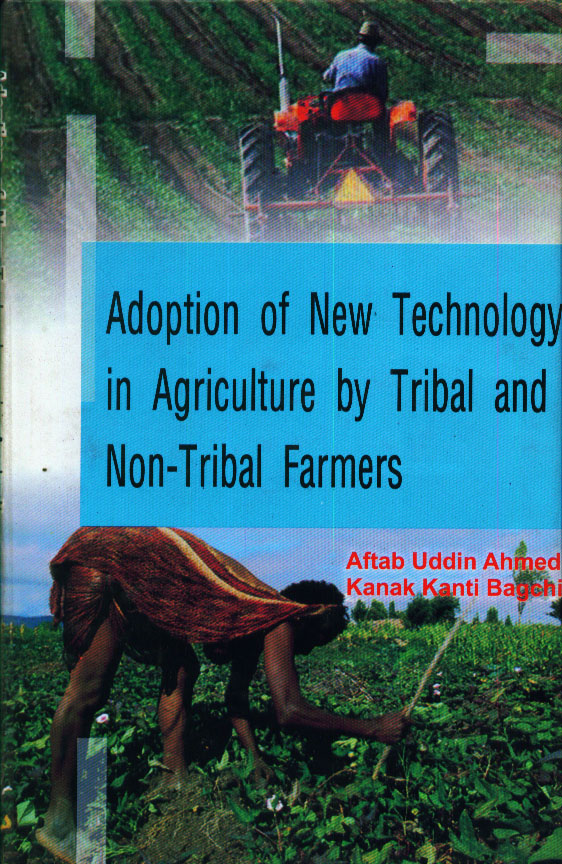
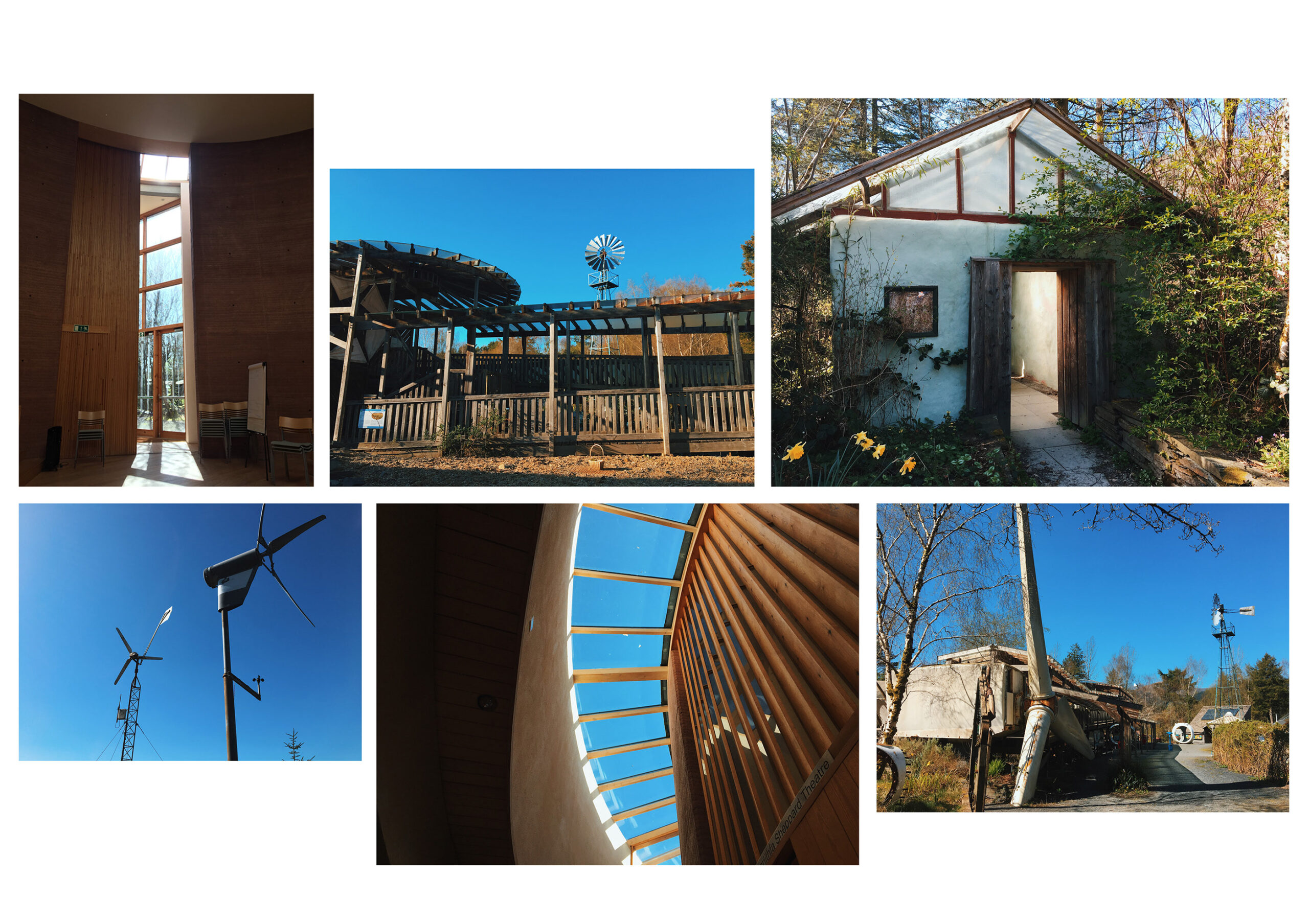
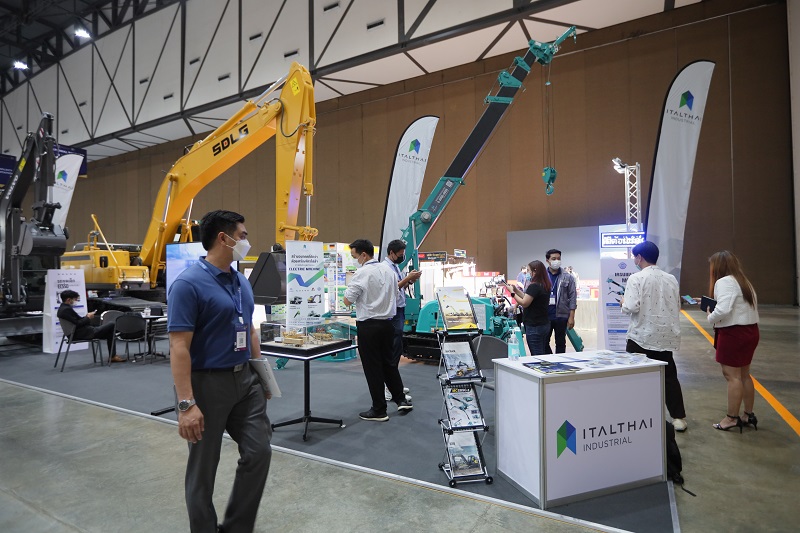
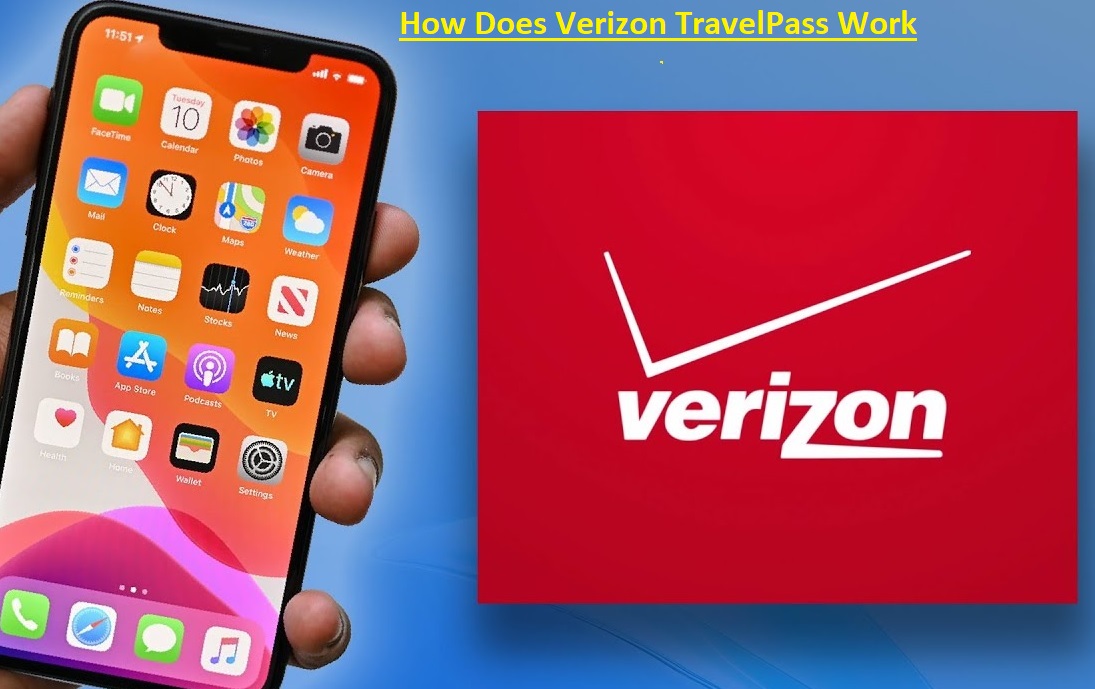
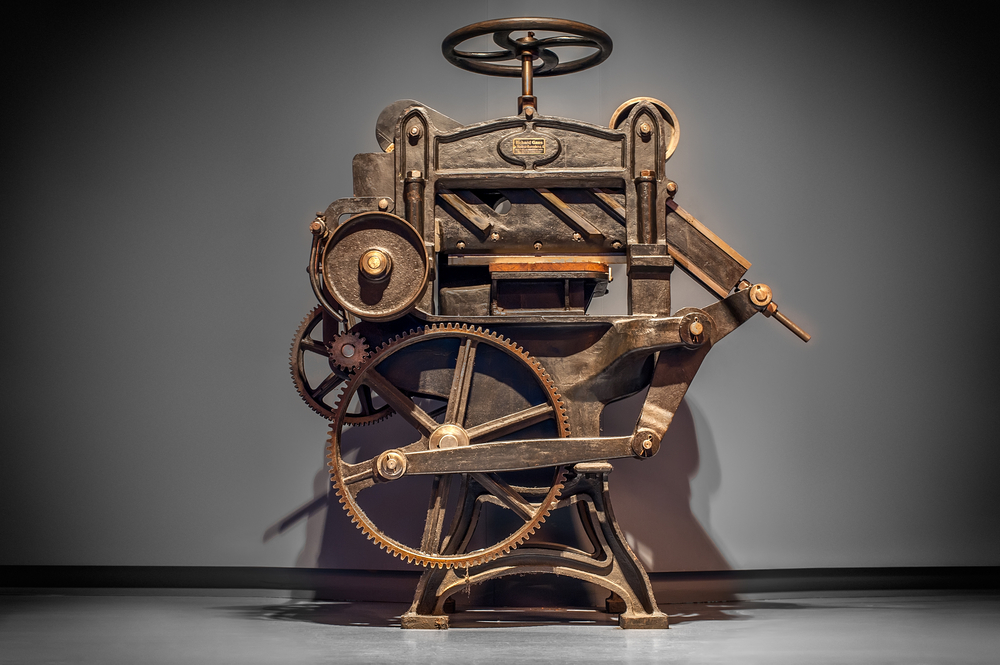
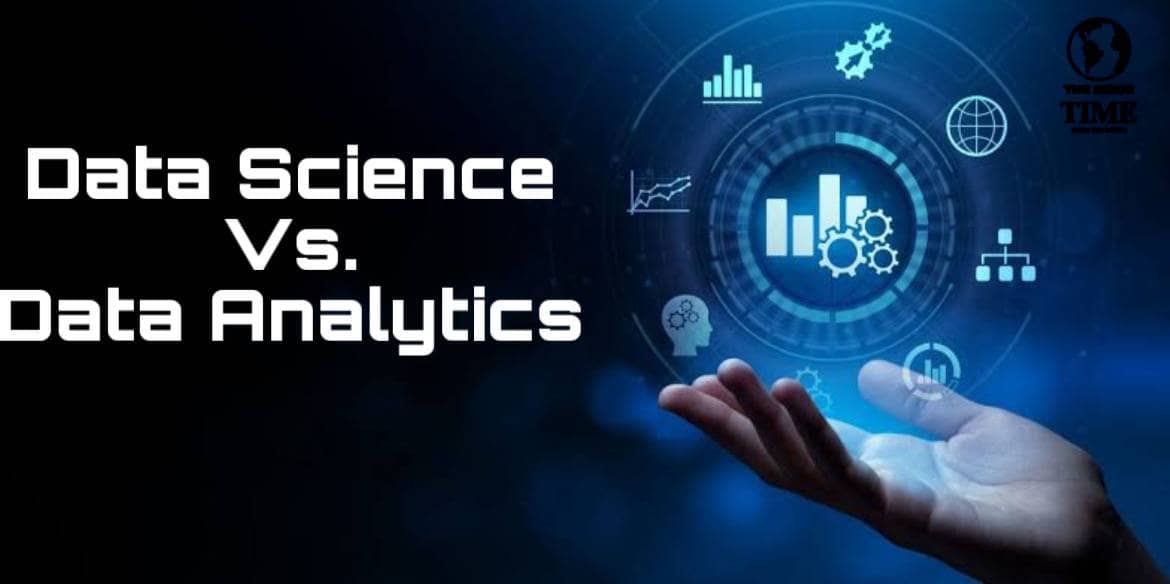

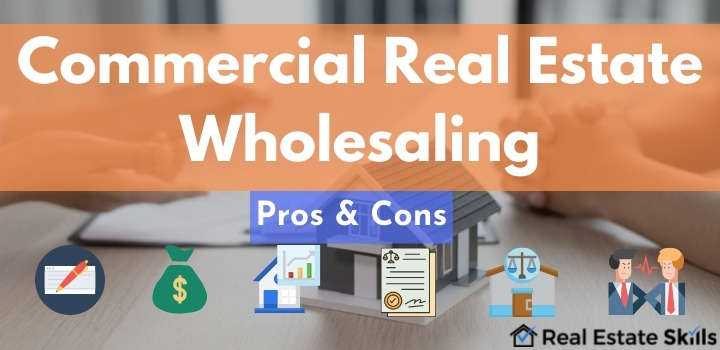
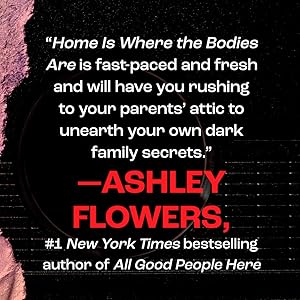
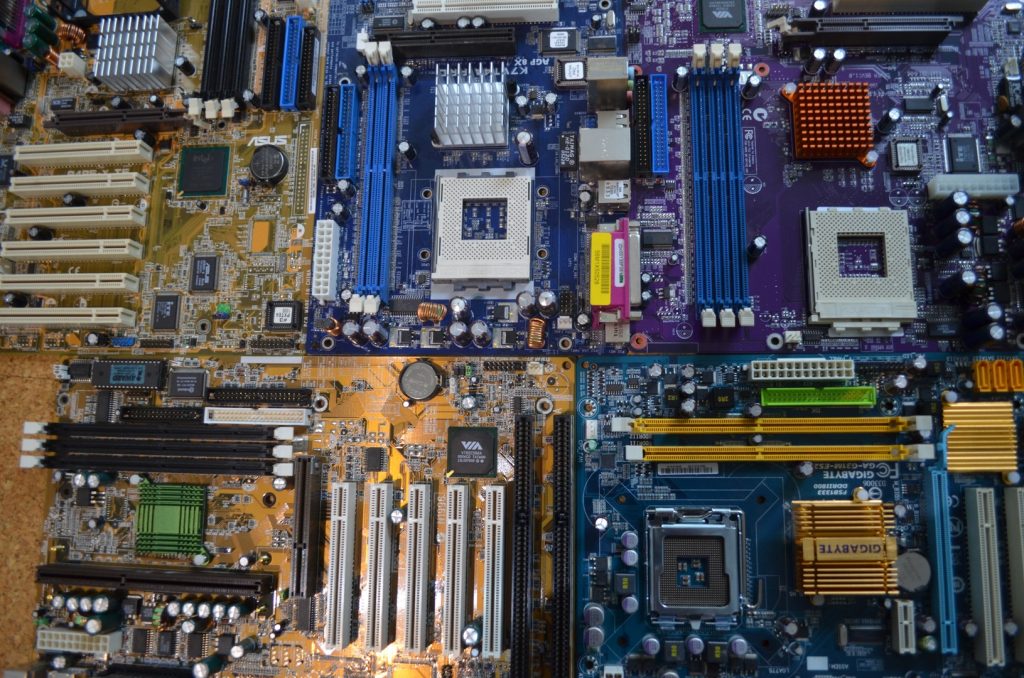